What is an Action Potential?
Dendrites on a neuron receive stimuli, but the soma and even the axon can receive stimulation. Stimuli can come from the environment (sound waves), internal visceral sensations, or other neurons. The stimuli that neurons receive will either increase or decrease the likelihood that a neuron will fire an impulse along its axon. Action potentials are the impulses neurons send along their axons.
Action Potential Vocabulary
Below are a few key terms you need to know to understand how an action potential works.
The membrane potential is the voltage along a neuron’s cell membrane, specifically the charge along the intracellular membrane. At rest, a neuron’s intracellular fluid has a negative charge, and the extracellular has a positive charge.

Depolarization to the threshold is when the membrane potential becomes more positive than the resting potential.


The threshold potential is the amount of depolarization required to fire an action potential. The average stimulus needed to reach the threshold potential is 15 mV.


Rapid depolarization (aka the overshoot)occurs when the membrane potential reverses its charge—i.e., inside the neuron becomes positive and outside the neuron becomes negative. Rapid depolarization is the product of the rapid influx of Na+ into the neuron.

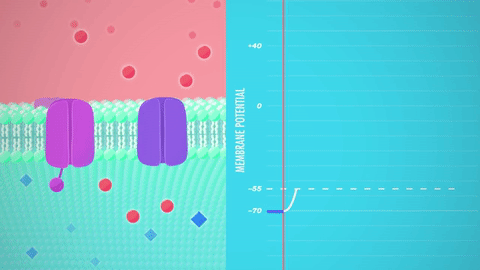
Repolarization occurs after rapid depolarization and returns the neuron to its resting membrane potential—i.e., the inside of the neuron becomes negative and the outside positive. The K+ out of the cell leads to the repolarization of the membrane potential.

Hyperpolarization (aka undershoot)happens when the membrane potential becomes more negative than the resting potential. Hyperpolarization occurs when too much K+ leaves the neuron.

The Absolute refractory period happens when a neuron’s electrical potential exceeds its concentration potential by such a degree that no stimulus can overcome its equilibrium potential. It begins with rapid depolarization and ends with repolarization.
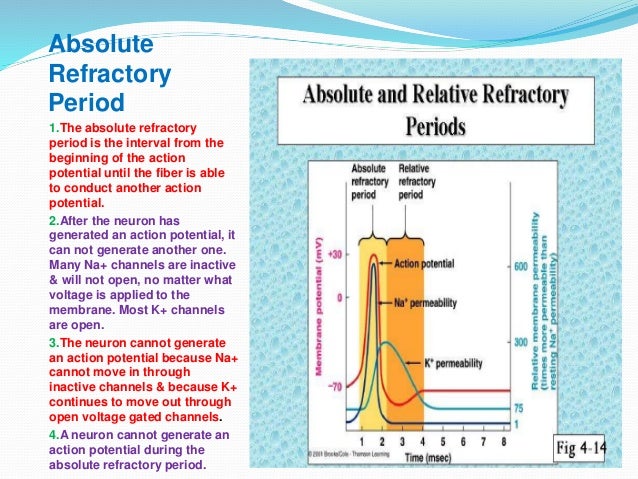
The relative refractory period starts with hyperpolarization and ends when the resting membrane potential reestablishes. During the RRP a neuron can fire an action potential if it receives a strong stimulus.
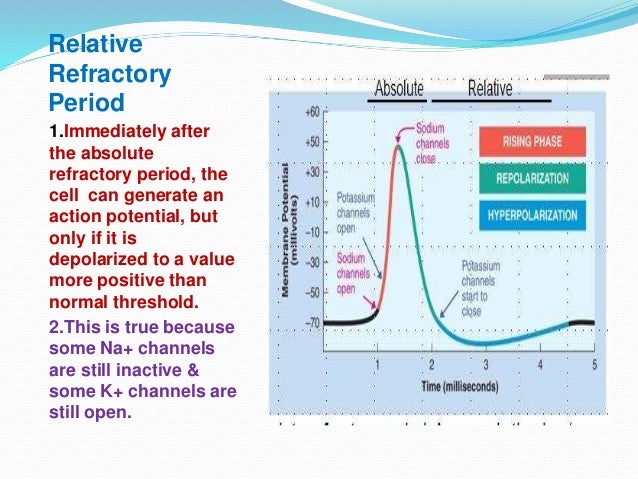
The All or None Event is the principle that governs whether a neuron will fire an action potential. The two requirements for the all or none event are as follows:
- A neuron must reach its threshold potential to fire an action potential.
- A neuron that reaches its threshold potential will fire an action potential of the same strength and speed no matter the starting stimulus’s power. For example, if the stimulus required to reach the threshold potential is 15 mV, then a stimulus of 15 mV will lead to an action potential of the same strength and speed as a 20 mV stimulus.
- However, a stronger stimulus will increase the frequency of action potentials.
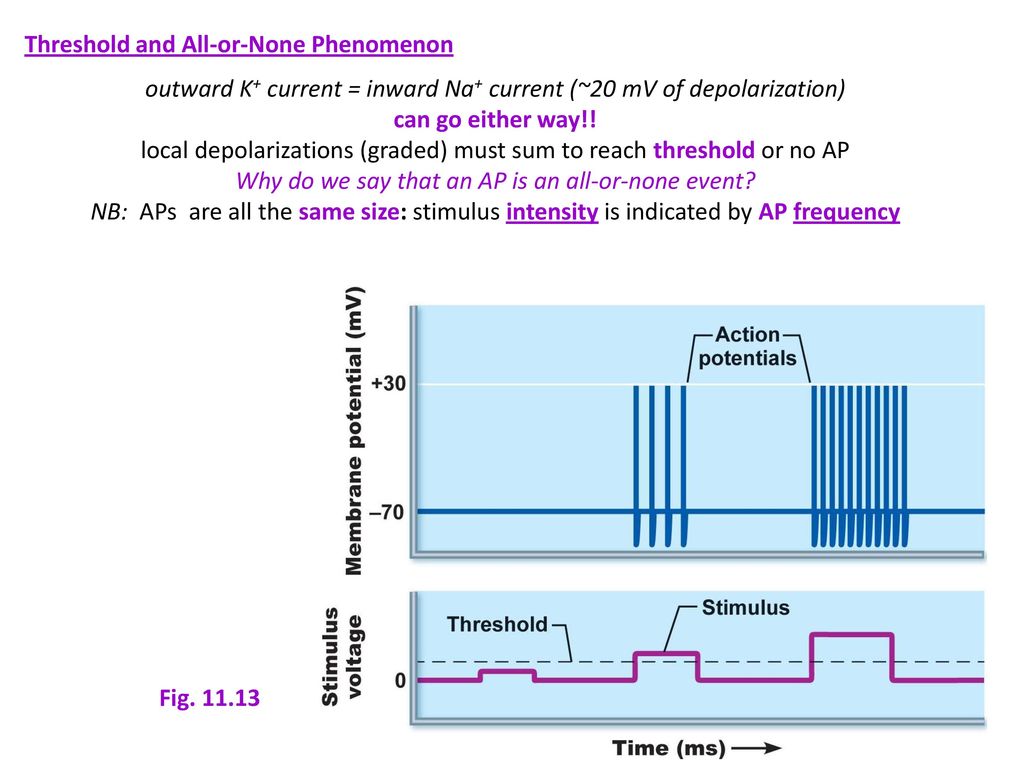
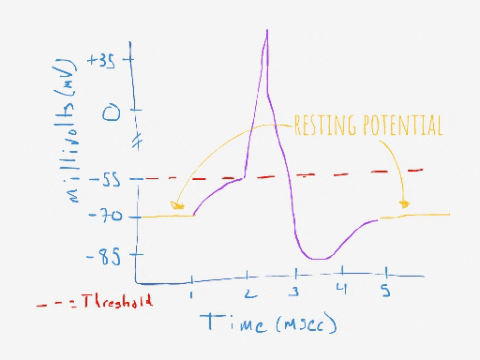
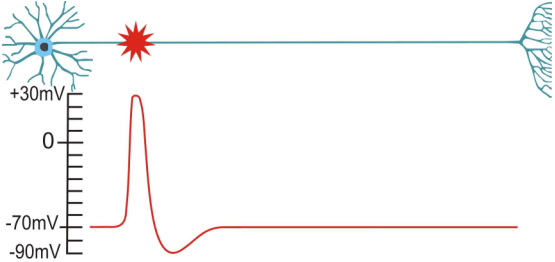
An Overview of Action Potential Physiology
The axon hillock’s job is to calculate the value of the neuron’s stimuli from the environment or other cells. Summation occurs when the axon hillock calculates the voltage of the incoming negative and positive stimuli. If the stimuli’ summation is 15 mV (threshold potential) or greater, a neuron will fire an action potential. Any summation less than the threshold potential will not lead to an action potential. Note: An EPSP and an IPSP are types of stimuli.
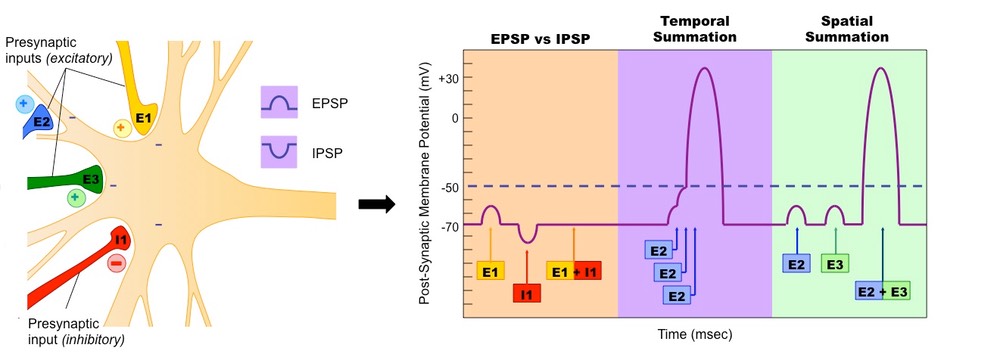
First, let’s start with a simple step-by-step explanation of an action potential.
- The Na+/K+ pump maintains the resting membrane potential.
- Threshold potential occurs when the summation of stimuli reaches an average of 15 mV.
- Rapid depolarization begins, Na+rushes into the neuron, and the membrane potential reverses—the inside becomes positive and the outside becomes negative, and the absolute refractory period begins.
- Repolarization begins when Na+ stops entering the cell, and K+ leaves the neuron—the inside becomes negative and the outside positive. The absolute refractory period ends with repolarization.
- Hyperpolarization begins when too much K+ leaves the cell, and the membrane potential becomes more negative than the resting potential. During hyperpolarization, the relative refractory period begins.
- Resting membrane potential reestablishes, and the relative refractory period ends.
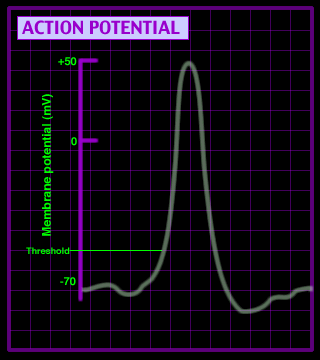
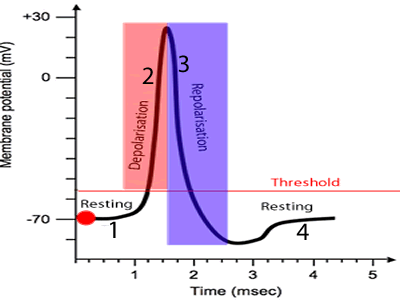
How Channel Proteins Govern Action Potential Physiology
A neuron’s cell membrane has sodium-specific channel proteins and potassium-specific channel proteins. When a neuron is at resting potential, none of the Na+ channel proteins are open, and most of the K+ channel proteins are not open. The positive charge of the protein channel wall is what keeps the channels closed during resting potential. At resting potential, the positive extracellular fluid repels the positive sides of the Na+ channel protein, closing the channel protein. But, when the extracellular fluid gains a positive charge, the repulsion of the Na+ flips, opening the channel protein. K+ channel proteins act similarly. (See the below figure.)
The following seven steps summarize the diffusion of Na+ and K+ ions during an action potential.
- Resting-State
- Na+ and most K+ channel proteins are closed
- A negative charge inside the neuron and a positive charge outside the neuron
- Na+/K+ pump pumps Na+ ions that “sneak” into the neuron via leaky Na+ channel proteins back outside the neuron
- Na+/K+ pump pumps K+ ions that “sneak” out the neuron via leaky K+ channel proteins back inside the neuron
- Depolarization to Threshold
- The axon hillock calculates the stimuli it receives from the dendrites—summation
- If the summation of the stimuli moves the charge of the membrane potential in the positive direction, then a few Na+ transport proteins open
- Na+ diffuses into the neuron, depolarizing it
- If enough Na+ enters the neuron, the threshold occurs, and rapid depolarization will begin
- Rapid Depolarization
- All Na+ transport proteins open and Na+ ions enter the neuron
- The influx of positive Na+ ions causes reverse the membrane potential, and the inside of the neuron becomes positive, and the outside of the neuron becomes negative
- Repolarization
- All the Na+ transport proteins close; no more influx of Na+
- Na+/K+ pump pumps Na+ out of the neuron
- K+ transport proteins open and K+ leaves the neuron
- The efflux of K+ ions reverses the membrane potential, intracellular fluid becomes negative, and the outside becomes positive.
- Hyperpolarization
- K+ transport proteins are slow to a close, so too much K+ leaves the neuron; inside becomes more negative than the resting potential
- The closure of the channel proteins and the Na+/K+ pump helps reestablish resting potential
- Absolute Refractory Period
- The absolute refractory period (ARP) begins with rapid depolarization and stops at the end of repolarization
- Na+ channel proteins are inactive during the AFP; so, it does not fire a new action potential
- The ARP ensures that action potentials will always travel in one direction down an axon
- Relative Refractory Period
- The relative refractory period (RRP) starts at hyperpolarization and ends with the re-establishment of the resting membrane potential
- During the RRP, the membrane potential is more negative than the resting potential, but a strong stimulus can fire a new action potential. For example, let’s say the resting membrane potential is -70 mV, and the threshold potential is -55 mV. At resting potential, the axon hillock needs to receive a 15 mV sum to reach the threshold potential and fire an action potential. During the RRP, the membrane potential may be -85 mV, but it still sets the threshold potential at -55 mV. The axon hillock needs to receive a 30 mV sum to reach the threshold potential and fire an action potential.


Action Potential Speed
The larger the axon diameter and the more myelin surrounding the axon, the faster the action potential. For example, an action potential may travel down an axon with a small diameter and no myelin at a rate of less than one meter per second. Contrary, action potentials can reach speeds of over one hundred meters per second along axons with large diameters and are surrounded by a thick layer of myelin.

Salutary conduction is the term that describes the propagation of an action potential along a myelinated axon. An action potential appears to leap from one node of Ranvier to the next as it travels along a myelinated axon. But, the actual movement does not involve the leaping of an action potential. Instead, the movement of Na+ and K+ only occurs at the nodes of Ranvier. The nodes of Ranvier lack myelin but contain Na+ and K+ channel proteins. It is at the nodes that the action potential recharges. The lengths of the axon membrane covered with myelin do not have protein channels. Myelin insulates the axon, and the current (action potential) moves through the axon’s myelinated part. The action potential recharges at the next node of Ranvier, and the process repeats until the action potential reaches the end of the axon.
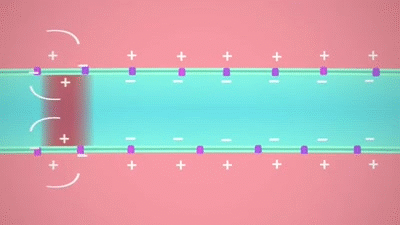
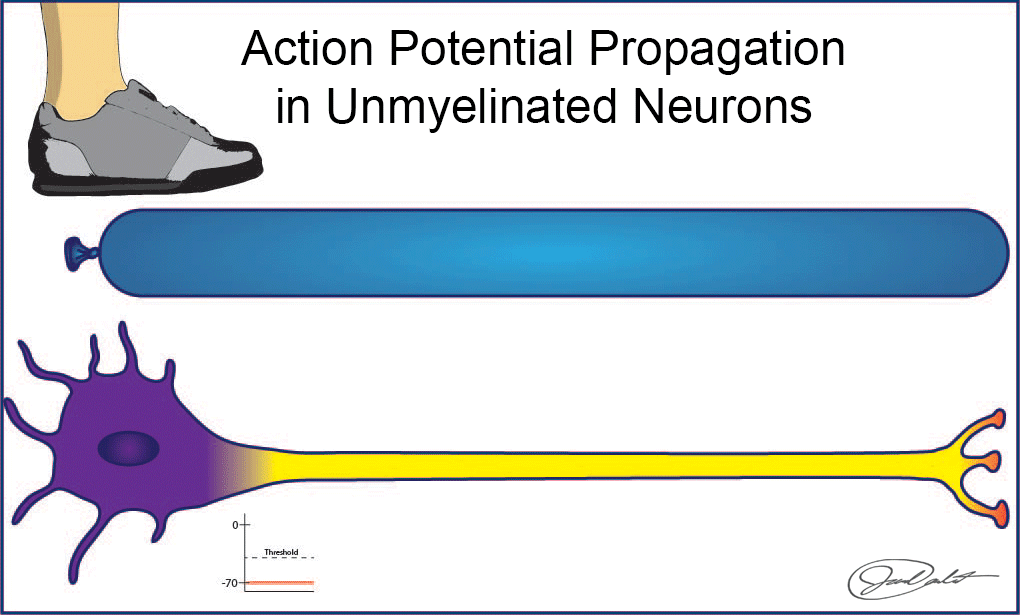
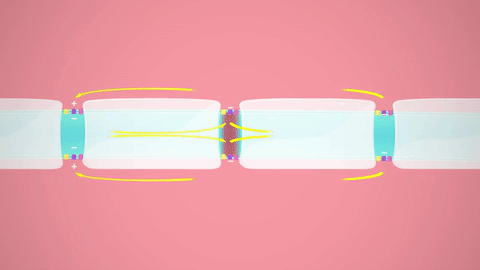

Graded Potentials: Action Potential Stimuli
Graded potentials are electrical currents that travel down the dendrites toward the axon hillock. Graded potentials spawn from a stimulus a dendrite receives from the environment or another cell. Like action potentials, graded potentials depend on the permeability of Na+ and K+ along a neuron’s membrane. But, action potentials and graded potentials have two primary differences.
- Graded potentials have no threshold potential, so any sized stimulus can start a graded potential
- The strength of a graded potential fades as it travels down a dendrite. Myelin does not cover dendrites, and dendrites’ membranes contain fewer Na+ and K+ channels. So, dendrite membranes have less resistance and more leakage.
Dendrite diameter affects the velocity of a graded potential. The larger the diameter, the faster the graded potential.
There are two types of graded potentials. Excitatory graded potentials depolarize a neuron’s membrane, making it more likely to reach its threshold potential. Inhibitory graded potentials hyperpolarize a neuron’s membrane, making it less likely to reach its threshold potential.
Graded Potentials vs. Action Potentials
Action Potential | Graded Potential | |
---|---|---|
Has a Threshold Potential | Yes | No |
Strength remains constant | Yes | No |
Travels along the Axon | Yes | No |
Travels along the dendrites and soma | No | Yes |
The diameter of a(n) axon/dendrite affects impulse speed | Yes | Yes |
Does myelin affect its speed? | Yes | No |
Click here to visit an awesome website explaining action potentials and graded potentials
Chapter Summary

Steps 1-8 below explain each of the eight steps in the above figure.
- The neuron receives a net positive charge at the axon hillock, which opens a few Na+ voltage transport proteins. Na+ begins to diffuse into the axon, which depolarizes the membrane potential.
- If the net positive charge reaches +15 mV, all the Na+ transport proteins open, and Na+ rushes into the axon. The influx of Na+ causes the axon’s inside to become positive and the outside of the axon to become negative. This is called rapid depolarization.
- The sodium transport protein gates rapidly close, preventing Na+ from diffusing into the cell. Rapid depolarization has ended, and the cell enters the absolute refractory period where the cell cannot fire a new action potential. The absolute refractory period prevents multiple, simultaneous action potentials and ensures the action potential travels in one direction down the axon toward the terminal endings.
- The K+ transport proteins finally open, and K+ diffuses out of the cell. The efflux of K+ causes the inside to become negatively charged and the outside to become positively charged. This is called repolarization.
- During repolarization, the sodium/potassium pump pumps the Na+ that diffuses into the cell during rapid depolarization out of the cell.
- The K+ transport proteins are slow to close, so too much K+ diffuses out of the axon. This causes the inside of the neuron to become more negative than the resting potential. This is known as hyperpolarization.
- All of the K+ transport proteins close, and the sodium/potassium pump pumps the K+ that diffuses out of the cell during repolarization/hyperpolarization back into the cell.
- Resting Potential is re-established.